Technology Trends for Future Radar Systems
Despite the widespread availability of advanced passive sensors, which do not emit electromagnetic signals detectable by the enemy, radar remains the main sensor of modern combat aircraft. Modern radar systems offer significant performance and their associated technologies are constantly evolving.
There are still some persistent unsatisfactory features, however, frequently stemming from trade-offs between design and cost, which are the objects of continuous investigation by designers, including:
- Some radars emit identical signals;
- Some transmit on only one frequency at a time;
- Some see only a limited area at any one time; and
- Many are still subject to distortion and other aspects of signal degradation.
For these and other reasons, including operational and practical considerations, the principal requirements for future radar are:
- High flexibility in signal generation and processing;
- New digital components for advanced processing;
- Advanced software;
- Efficient time-frequency-space coverage;
- Simultaneous air-to-air and air-to-surface operations;
- Low sensitivity to electronic countermeasures (ECM) and new advanced (electronic counter counter measures) ECCM capabilities;
- Advanced communications and data sharing capability;
- Ability to provide tactical advantages in the future net-centric battlespace;
- Small volume and light weight; and
- Acceptable cost.
It would appear that the limitations of current state-of-the-art radar systems are likely to be overcome in the next decade, thanks to advanced concepts which include: Intelligent signal coding, multiple Input Multiple Output (MIMO) technology, digital beamforming, array imaging, and RadCom (radar and communication) techniques.
Radar Signal Coding Requirements
One of the most important requirements for future radar is for separate coding of each transmitted signal. This requires signal compression in both time and frequency: For military applications, the detectability of this kind of signal for localisation and use of countermeasures becomes very difficult, due to their similarity to certain communication signals. For small radars, as fitted, e.g. to UAVs, this solution also increases power efficiency. Generally, signal coding selection has to consider the following characteristics: Simplicity of signal generation, processing and compression; ability to transmit information; compatibility with MIMO operations; and simple hardware design.
Several different coding schemes exist, such as CDMA (Code Division Multiplex Access), DSSS (Direct Sequence Spread Spectrum), and OFDM (Orthogonal Frequency Division Multiplexing). OFDM coding features several of the necessary capabilities, such as good decorrelation, simple processing and ease of realisation.
MIMO Technology
A technology originally developed in communications science to improve fundamental capabilities such as coverage, data rate, and signal characteristics (which are the same enhancements that future radars require), MIMO technologies have been intensively researched in recent years. MIMO radars radiate uncorrelated signals simultaneously in multiple directions or in a single direction with orthogonal polarisation. This improves coverage and the quality of the signal return quality in ECM conditions. Decorrelation between the transmitted signals is achieved by OFDM, which negates degradation in radar range resolution. The decorrelation of each transmitted signal is essential, since small, remote targets might otherwise not show up on the radar image. MIMO radar employs spatial multiplexing to split the transmitted signal into multiple sub-signals, and transmits each of them, uncorrelated, in a different beam or via different sub-channels. MIMO is a system of multiple antennas, each radiating a signal independently of the others. Due to different waveforms, the echo signals can be re-assigned to the single transmitter, thus creating a virtual enlarged receive aperture. The transmit structure of MIMO radar is characterised by the following features: Spectrally interleaved multicarrier signals; relative orthogonal positioning at each transmitter; and bandwidth for each transmitter to maintain Doppler resolution and avoid range degradation.
Bandwidth and range resolution are not affected, but require time and frequency synchronisation of transmitters. MIMO optimises wave propagation and signal processing in both communications and radar and offers multiple functionality, including multiple targets, 3D, interference reduction, and multi-path and signal-to-noise ratio (SNR) optimisation. The parallel mode sub-channels have to be highly de-correlated in time, frequency, or code-multiplexing. MIMO radars are characterised by improved spatial resolution and can also provide improved immunity to interference, clutter and jamming, while an increase in SNR increases target detection probability of detection of the targets. These systems can classify a very high number of targets by irradiating them with multiple individual waveforms. MIMO architecture provides a set of transmit and receive antennae that can view the target from different angles. The current challenge is the search for orthogonal waveforms, so as to remove interference problems between the antennae during both the transmission and pulse compression phases.
New operational scenarios demand greater flexibility and higher capacity detection for radar systems. In this context, Noise Radar Technology (NRT) allows the radar to transmit signals originating in a noisy generation process. Their nature gives these signals statistical characteristics always known in advance. The current challenge in this field is to design an architecture that can generate, with maximum reliability, specific waveforms with special properties for the use in radar detection, orthogonal to each other and in very high numbers. Combining MIMO radar requirements and NRT technology would mean being able to generate a large number of complex waveforms whose functions of auto-correlation and cross-correlation present the lowest possible lobes, with specific attention paid to a possible reduction in signal power due to the transmitter not transmitting at peak efficiency in specific conditions.
Digital Beamforming
Conventional methodologies limit the effectiveness of radar scanning. One solution is DBF (Digital Beamforming), in which multiple independent beams are formed by an array of antennae, with the advantage that beam coverage can be simultaneously processed. Beamforming is the combination of radio signals from a set of small non-directional antennae to simulate a large directional antenna. This can be pointed electronically, although the antenna does not physically move. In radar applications, beamforming can be used to steer an antenna to determine the direction of the target. In digital beamforming, phase shifting and amplitude scaling for each antenna element are made digitally and the received results fused. This digital processing requires that the signal from each antenna’s element is digitized by an A/D (Analog-to-Digital) converter. The ability to measure the angle of the return signal with higher resolution, which requires accurate measurement of signal phases, from which the angle of arrival is calculated, is termed ‘super-resolution’.
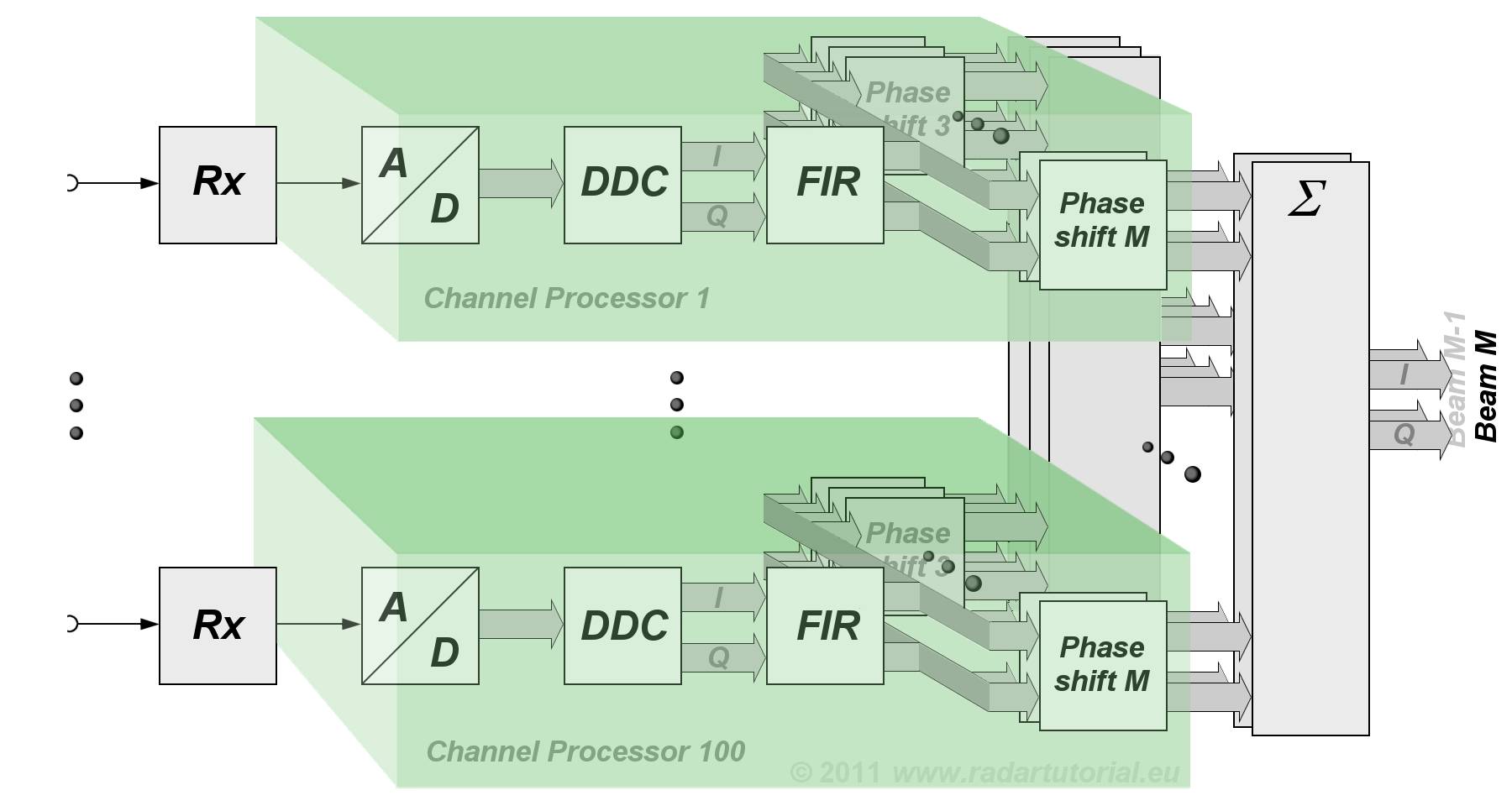
RADCOM
The concept of combining radar and communication systems on a single platform is not new, but because of the significant differences between the two systems, has not yet been fully developed. The major obstacle was that radar and communication systems use very different frequency ranges; thanks to modern digital processing, however, the gap between hardware requirements for radar and communications systems has narrowed, and synergy between the requirements can be achieved. Integrated RADCOM systems share hardware resources and the full spectrum to fulfil both functions simultaneously. An integrated RADCOM system not only alleviates the problem of available electromagnetic spectrum, but also reduces weight and volume of the relative avionics by reusing the hardware. Today OFDM can be applied to MIMO radars to achieve both capabilities. The choice of OFDM for common RADCOM signals offers interesting advantages such as resistance to specific signal distortion (multipath fading) and a simpler approach to synchronisation. This solution provides:
- Simultaneity due to OFDM-based transmit signals;
- Multi-user capability without interference;
- Reduced number of components required;
- Higher spectral efficiency;
- Higher power efficiency; and
- Capacity for advanced on-board data fusion.
Reliability of Kelvin Hughes SharpEye Technology
Earlier this year, Kelvin Hughes, a world leader in the design and supply of navigation and security surveillance systems, has made several strides in selling its SharpEye radar systems.
SharpEye is available in both I (X) and E/F (S) frequency bands, sharing a high degree of component commonality due to their similar modular design and system architecture. Kelvin Hughes’ ability to offer variants of SharpEye across both frequency bands to a similar design offers the international community the opportunity to realise significant savings in training, documentation and through life support.
The SharpEye solid-state radar transceiver transmits a low power, patented pulse sequence incorporating pulse compression that enables superior range discrimination across all range scales. This means that multiple operators can have access to an optimum picture at both long and short ranges simultaneously. Doppler processing of radar returns provides coherent information concerning a target’s velocity and improves the probability of detection of small objects with a low RCS (Radar Cross Section). Through a series of electronic filters, SharpEye is able to distinguish between targets of interest and clutter whilst customisable waveforms can be configured for specific threats and to enhance the detection of specific targets of interest such as UAVs and helicopters.
The radar’s low power output also reduces the probability of detection by enemy ESM equipment.
With its advanced target detection capability, the solid-state SharpEye radar also provides great levels of maritime situational awareness. Through the Doppler processing of the radar returns, SharpEye can identify semi-submersed objects to the smallest of uncooperative craft, even in the most severe of weather conditions. Moreover, the radar’s low power output reduces the probability of detection by ESM equipment and, with no magnetron involved, offers significant through-life cost benefits. Maintenance requirements are reduced to a minimum and there is no need for any formally qualified radar engineer on-board.
Coming back to contracts, Rodman Polyships has contracted Kelvin Hughes to supply X and S band SharpEye radar systems for a range of new vessels being supplied to the Royal Oman Police Coast Guard (ROPCG).
When equipped with SharpEye, with its greatdetection performance, the vessels will be ideally suited to patrol the full extent of the Omani coastline which runs for some 1,700km from the Strait of Hormuz in the north to the borders of the Republic of Yemen in the south-west.
Furthermore, working in association with <rot>Lockheed Martin Integrated Systems UK,<P> who have signed a £44 million contract with the UK MoD, Kelvin Hughes will be supplying SharpEye systems for the Royal Navy’s (RN) Type 23 frigates, HUNT and SANDOWN-class Mine Counter Measure Vessels, RFA support vessels, and fast patrol boats, as well as the ASTUTE, TRAFALGAR, and VANGUARD-class submarines. The contract with Lockheed Martin also includes options for the introduction of navigation radar systems for future RN platforms such as the QUEEN ELIZABETH carriers, the Type 26 Global Combat Ship, and SUCCESSOR submarines.
Further Technology Developments
A prototype of a quantum radar has been developed by the University of York. This technology has the potential to detect objects invisible to conventional radar systems, thanks to hybrid technology that uses quantum correlation between microwave and optical beams to detect objects with low reflectivity, such as stealth aircraft. The system operates at much lower energies than conventional radar, and is characterized by a special converter able to couple the microwave beam to an optical beam using a nanomechanical oscillator. This device can generate microwave-optical signals during emission, or convert a microwave into an optical beam during acquisition of the reflection signal from the target. A conventional radar antenna emits a microwave to scan a region of space. Theoretically, any target would reflect the signal to the source, but objects characterised by low reflectivity in a specific region, with high background noise and/or high levels of ECM, are notoriously difficult to detect using a traditional system. Quantum radar operates more effectively and exploits quantum signals to enhance sensitivity and detect small return signals from a very noisy environment. Further studies are being directed towards a new technology known as passive radar, considered one of the emerging technologies in military radar applications. While traditional radar emits electromagnetic signal in operation, passive radar is able to use existing electromagnetic signals from the atmosphere to support imaging and tracking capabilities. This kind of radar is also less expensive and the market is expected to be worth some US$10 billion in annual spending by 2023.
Metamaterials (advanced materials with properties that not found in nature) may be the next improvement in radar technology. Research and development using enhanced materials can drastically reduce size, weight and price of radar devices. With these physical, operational and cost shifts, metamaterials-based radar systems could be applied to mini-UAVs. The technology promises significant cost savings for these kinds of sensors, allowing very advanced radars to be produced for a few thousand dollars once the technology has been matured.
Improvements in radar systems are not only taking place on the hardware-digital side. The enhanced transmit/receive and computer-processing technologies of modern radar system are also giving rise to imaging radar system that produce high-resolution pictures from return signals. Signal-processing designers are working on an advanced technology known as ‘circular synthetic aperture radar’ (also known as circular SAR and video SAR). This technology allows for a 3D image based on successive layers of radar data. Although radar imaging is not a new technology, it is now possible to obtain high-quality images in real time, since processing time is less than aperture time and image generation is very close to real time.
The next decade will be a very important period in radar computing and for avionics in general. For radar systems increasing demands will continue for processing power in the following fields: wideband operation, sensor fusion, cognitive sensing, platform networking, autonomy, passive capabilities, artificial intelligence. Wideband multifunction operations in particular will maximize some fundamental functions relative to digital beamforming for multi-sensor applications. This also imposes a further need for wideband frequency agility and offers an opportunity for much greater integration between radar, ECM/ECCM, and signal intelligence.
Both quantitative and qualitative aspects of sensor fusion will also change significantly in the future. This is due both to improvements in radar characteristics and relative processing power, and to the increase in data volumes emanating from networked platforms, as well as advanced requirements to provide data to systems operating as expert or artificial intelligence systems, such as the Artificial Neural Network (ANN).
In conclusion, future radar will: Be completely digital; transmit/receive coded signals; operate in an MIMO mode; have wider simultaneous coverage; have a virtually increased array size; work with an unlimited numbers of targets; be mono-, bi- or multi-static; be achieved with metamaterials; transmit information and communications; be more energy efficient; and be cheaper and smart.
Paolo Quaranta